An ammeter is an instrument for measuring electric current flow, calibrated in amperes. There are two main types of ammeter architectures: shunt ammeters and feedback ammeters.
Shunt vs. Feedback Ammeters
Shunt ammeters are the most common type and work in many applications; feedback ammeters are more appropriate when measuring small currents;their use is growing because the typical magnitude of the test currents used today is decreasing. However, choosing the proper ammeter depends not only on the magnitude of the current, but also on characteristics (most typically, the impedance) of the device under test (DUT).
Shunt Ammeters: DMMs
Shunt ammeters are the most common ammeter type and are found in almost all digital multimeters (DMMs). These meters measure current by developing a voltage at the input terminal that is proportional to the current being measured (Figure 1).
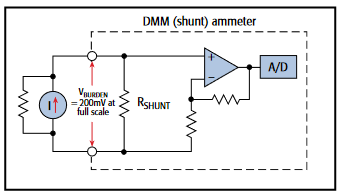
The main drawback associated with shunt ammeters is their fundamentally high input impedance design. This drawback becomes more significant with decreasing current, because a larger shunt resistor must be used in order to develop a measurable voltage. However, as long as the shunt resistor is significantly smaller than the resistance of the DUT and the currents to be measured are not very small (not much lower than microamp level [10-6A]), shunt ammeters work fine.
Voltage Burden
The terminal voltage of an ammeter is called the voltage burden. This voltage burden developed across the meter could result in significantly lower current through the load than before the meter was inserted, therefore, the ammeter can't read the current it was intended to measure.
An ideal ammeter would not alter the current flowing in the circuit path, so it would have zero resistance and zero voltage burden. A real ammeter will always introduce a non-zero voltage burden. In general, the error term caused by an ammeter is stated as the ammeter's voltage burden divided by the resistance of the DUT. A shunt ammeter's voltage burden is typically on the order of hundreds of millivolts
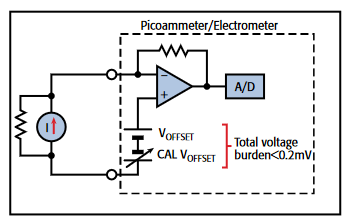
Feedback Ammeter
Feedback ammeters are closer to "ideal" than shunt ammeters, and should be used for current measurements of microamps or less (10-6A) or where it is especially critical to have an ammeter with low input impedance. Instead of developing a voltage across the terminals of the ammeter, a feedback ammeter develops a voltage across the feedback path of a high gain operational amplifier (Figure 2). This voltage is also proportional to the current to be measured; however, it is no longer observed at the input of the instrument, but only through the output voltage of the opamp. The input voltage is equal to the output voltage divided by the op-amp gain (typically 100,000), so the voltage burden has now typically been reduced to microvolts. The feedback ammeter architecture results in low voltage burden, so it produces less error when measuring small currents and when measuring currents generated by low impedance devices. Keithley electrometers and picoammeters employ feedback ammeter technology.
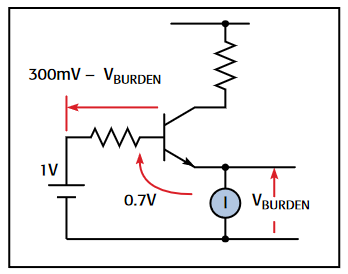
Figure 3 illustrates the problems caused by high voltage burden when measuring the emitter current of a transistor. Even though the basic current measurement could be well within the measuring capability of the DMM, the DMM's voltage burden significantly reduces the voltage applied to the DUT, resulting in ower measured emitter current than intended. If a picoammeter or electrometer were used instead, the voltage burden would cause a negligible change in emitter current.
Sources of Generated Current Error
Low current measurements are subject to a number of error sources that can have a serious impact on measurement accuracy. All ammeters will generate some small current that flows even when the input is open. These offset currents can be partially nulled by enabling the instrument current suppress. External leakage currents are additional sources of error;therefore, making properly guarded and/or shielded connections is important. The source impedance of the DUT will also affect the noise performance of the ammeter. In addition, there are other extraneous generated currents in the test system that could add to the desired current, causing errors. The following paragraphs discuss various types of generated currents and how to minimize their impact on the measurements.
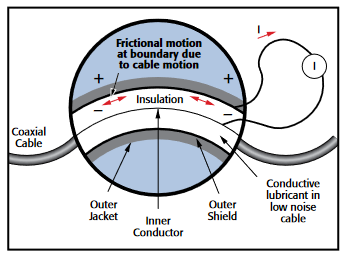
Triboelectric effects are created by charge imbalance due to frictional effects between a conductor and an insulator, as shown in Figure 4. Keithley's low noise cables greatly reduce this effect by introducing an inner insulator of polyethylene coated with graphite underneath the outer shield. The graphite provides lubrication and a conducting equipotential cylinder to equalize charges and minimize the charge generated.
Piezoelectric currents are generated when mechanical stress is applied to certain crystalline materials when used for insulated terminals and interconnecting hardware. In some plastics, pockets of stored charge cause the material to behave in a manner similar to piezoelectric materials. An example of a terminal with a piezoelectric insulator is shown in Figure 5. To minimize the current due to this effect, remove mechanical stresses from the insulator and use insulating materials with minimal piezoelectric and stored charge effects.
Contamination and humidity can produce error currents, which arise from electrochemical effects that occur when contaminants (in the form of ionic chemicals) create weak "batteries" between two conductors on a circuit board. For example, commonly used epoxy printed circuit boards, if not thoroughly cleaned of etching solution, flux, oils, salts (e.g.,fingerprints) or other contaminants, can generate currents of a few nanoamps between conductors (see Figure 6). To avoid the effects of contamination and humidity, select insulators that resist water absorption and keep humidity to moderate levels. Also, keep all insulators clean and free of contamination.
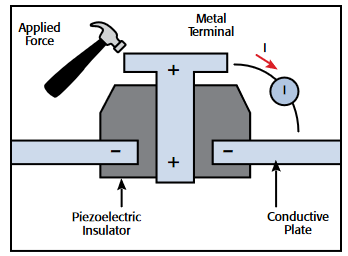
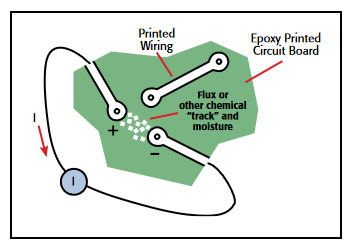
Figure 7 summarizes approximate magnitudes of the various currents.
High Resistance Measurements
For high resistance measurements (>1GΩ), a constant voltage is most often applied across the unknown resistance. The resulting current is measured from an ammeter placed in series, and the resistance can be found using Ohm's law (R= V/I).This method of applying a voltage and measuring the current (as opposed to applying a current and measuring the voltage), is preferred for high resistance measurements, because high resistances often change as a function of applied voltage. Therefore, it's important to measure the resistance at a relevant and controllable voltage. This method most often requires measuring low currents using an electrometer or picoammeter. All the low current techniques and error sources described in previous paragraphs also apply here.
Leakage currents are typical sources of error in high resistance measurements. They are generated by unwanted high resistance paths (leakage resistance) between the measurement circuit and nearby voltage sources; they can be reduced by employing proper guarding techniques, using clean, quality insulators, and minimizing humidity.
Typical resistance values of various insulating materials are shown in Figure 8. Absorbed moisture may also change the resistance of certain insulators by orders of magnitude. Table 1 shows a qualitative description of water absorption and other effects.
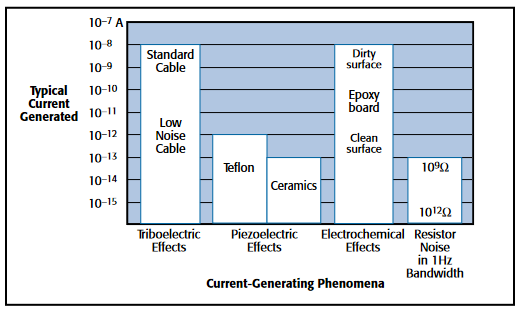
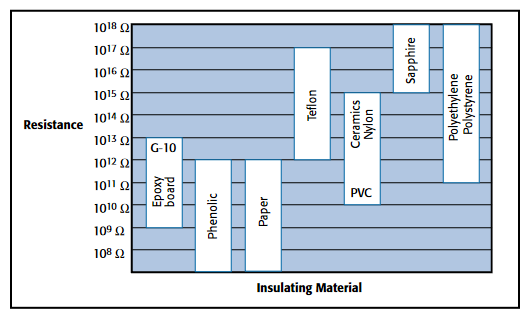
Alternating Polarity Method
When measuring materials with very high resistivity, background currents may cause significant measurement errors. They may be due to charge stored in the material (dielectric absorption), static or triboelectric charge, or piezoelectric effects.
The Alternating Polarity Method can virtually eliminate the effects of background currents in the sample. In this method, a bias voltage of positive polarity is applied, then the current is measured after a predetermined delay. Next, the polarity is reversed and the current is measured again, using the same delay. The polarity reversal process can be repeated any number of times. The resistance is calculated based on a weighted average of the most recent current measurements.
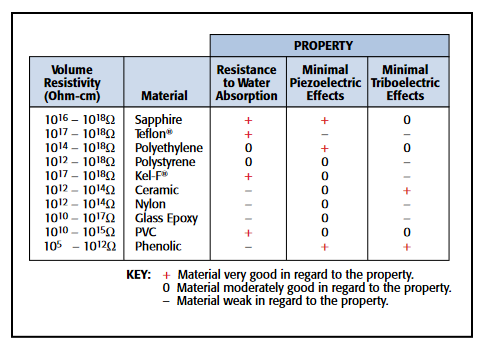