Introduction
In High Energy Physics (HEP) applications, the fundamental challenges facing operators and researchers are sustained maximum beam power and consistent beam stability in particle accelerators. To accomplish these objectives, operators need to be able to collect information on the spectral behavior of particle beams at the time of beam ramp up as well as during various experiments.
In this application note, the causes of beam instability and “blow up” - sudden total or partial loss of beam current - will be reviewed. We’ll discuss the advantages of using Real-time Spectrum Analysis to analyze and solve beam loss and instability problems versus the use of Swept Spectrum Analysis (SSA). And we’ll show how Tektronix real-time spectrum analyzers (RTSAs) are used to collect, analyze and present data in the High Energy Physics field.
Causes of Beam Instability and "blow up"
In a storage ring, particles (protons, electrons, or ions) are injected into a stable orbit that allows them to circulate for many hours. This orbit of particles is known as the “beam.” The beam is maintained in the storage ring at a certain velocity until such time as it is "kicked out" to the Linear Accelerator (LINAC). During the storage time, the beam’s velocity, path, coherence, and chromaticity are monitored and adjusted using magnets and RF fields. It is during this portion of the beam life cycle that it is most susceptible to errors and problems.
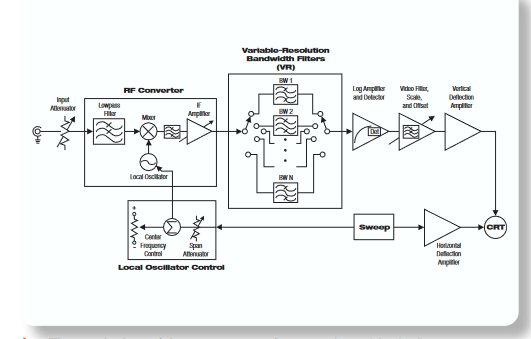
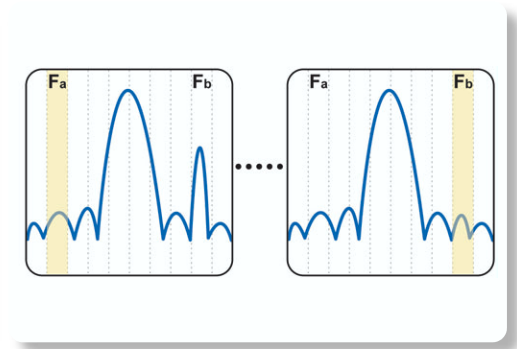
The beam orbit in the storage ring has design values called horizontal, vertical, and longitudinal “tune” frequencies, which are directly related to the beam’s position in the ring. The electrical characteristics of the focusing magnets and RF cavities around the ring determine these values.
Typically, particles are injected into the ring at low energy levels then “ramped up” to higher levels. During ramping, it is important that the horizontal and vertical tune frequen- cies do not shift, lest they hit upon a resonant combination that causes beam instability or sudden total loss of ring beam current (beam blow up).Beam instabilities can be caused by a number of factors. Non-linearities and/or differ- ent response times of independent controls such as beam position monitor (BPM) cables and circuits, magnets for guidance and focusing of the beam, Klystrons or Tetrodes (which provide power to RF cavities that transmit energy to the beam), and vacuum pumps and monitors can all cause beam instabilities. Vibrations and lack of proper shielding are other factors.
The challenge for operators and researchers is to correctly identify the factors causing beam instabilities and blow up so that costly accelerator time is not interrupted and experi- mental results are not compromised. The instrument most often used to identify problems in particle accelerator appli- cations is the spectrum analyzer. In the section that follows, we discuss the advantages of real time spectrum analyzers versus swept spectrum analyzers in HEP applications.
Using Spectrum Analyzers - Swept Frequency vs. Real Time
In swept frequency spectrum analyzers, signals are observed as a function of frequency – an approach that loses most, if not all temporal (time relative) information. Real-time spectrum analyzers allow the user to view and measure each portion of the input signal’s frequency spec- trum as a function of time. The differences, as they pertain to HEP applications, are as follows.
Swept Frequency Spectrum Analyzers
Figure 1 is a simplified block diagram of the swept frequency spectrum analyzer architecture. The local voltage-controlled oscillator continuously sweeps through a range of frequencies that are combined in a mixer stage with the input signal (a process known as heterodyning)
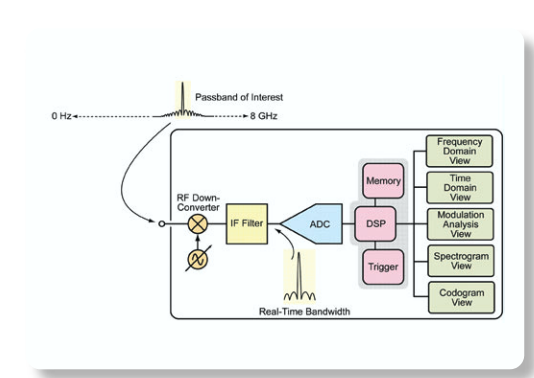
The mixer is a nonlinear device that produces the sum and difference of the two signals, along with the originals and their harmonics. The resulting signal components are sent through a resolution filter that extracts the desired mixing product. This signal product is then detected and sent to the Y-axis of the display (amplitude). At the same time the voltage controlled oscillator signal is processed and sent to the display to create the X-axis sweep (frequency).
The traditional swept spectrum analyzer makes amplitude vs. frequency measurements by sweeping a resolution bandwidth (RBW) filter over the frequencies of interest and recording the amplitude at each frequency point. While this method provides superior dynamic range, its disadvantage is that it only records the amplitude data in one frequency at a time. Sweeping the RBW filter over a span of frequencies takes time - on the order of seconds in some cases. A relatively stable, unchanging input signal is required.
If there is a rapid change in the signal, it is statistically probable that the change will be missed. As shown in Figure 2, the sweep is looking at frequency segment Fa while a momentary aberration is occurring at Fb. By the time the sweep arrives at segment Fb, the error has vanished. It does not get detected. There is no way to trigger on defined signal characteristics, nor is there a way to accumulate a record of longer-term signal behavior.
The response, or settling time, of the resolution filter limits the sweep rate of the analyzer. In addition, and sweep rate is proportional to the square of the bandwidth – the narrower the resolution bandwidth, the slower the sweep rate.
If the analyzer sweeps too quickly the displayed amplitude will be lower than the actual amplitude and the apparent frequency will be shifted to the right.
Theoretically, the sweep rate should be infinitely slow, but that is not realistic – so, some amount of acceptable error must be specified. Increased sweep times demand that the input signal remain stationary for even longer periods – usually disqualifying swept analyzers for the measurement of intermittent or periodic signals.
Traditionally the major advantages of swept-frequency analyzers over real-time instruments have been their dynamic range and frequency range. Recent advances in high-speed analog-to-digital (A/D) converter technology, however, have narrowed those advantages, as new real- time analyzers feature 70 dB or more of dynamic range and coverage from DC to 8 GHz – more than adequate for most HEP applications.
Real-time Spectrum Analyzers: Storing and Analyzing Frequency and Time
As time-varying signals become more common in HEP applications, the need for an alternative approach to RF acquisition and analysis becomes more urgent. The real-time spectrum analyzer has emerged to solve this tough measurement problem. Alone among the spectrum analyzer architectures, the RTSA can trigger on a frequency domain event, then capture and analyze any passband signal that falls within its real-time bandwidth.
Figure 3 depicts the RTSA architecture. An integrated down-converter positions the real-time bandwidth on any passband up to the analyzer’s upper limit. After filtering, the down-converted signal goes through an ADC that digitizes it, allowing triggering, capturing and analysis of the signal all at once.
Since the basic process is not one of sweeping across the RF input signal and building an image from serially acquired frequency steps, the RTSA’s digital IF architecture allows a continuous capture of “snapshots” known as frames. These frames accumulate in the memory as a seamless, continuous record of time.
The memory supports a variety of display and analysis tools including the spectrogram, which plots an entire series of frames to reveal signal changes over time. Thus the RTSA is the only RF signal analyzer optimized to produce a three dimensional display: frequency, power (amplitude), and time. If the passband of interest exceeds the real-time bandwidth of the RTSA, the RTSA can step through a series of frequency segments, just like the swept analyzer. In doing so, each sweep captures a band of frequencies equivalent to the RTSA’s real-time bandwidth. Then the instrument concatenates the frequency bands and presents a conven- tional frequency-domain display.
RTSA Measurements in Particle Accelerator Applications
Tektronix RSA2200A and RSA3300A Series Real-time Spectrum Analyzers are used in particle accelerator applications to monitor beam ramping, measure horizontal and vertical tune frequencies during ramp up; measure chromaticity; and monitor amplitude, frequency and phase modulations. In addition, the RSA’s allow the display of power vs. time information and provide a frequency mask trigger for monitoring beam stability.
Monitoring beam ramping
In the following example, we will monitor a beam ramp up that is typically 160 ms in duration, and compare that with the commissioning phase during which the beam may be ramped up at a rate of 1 second. We will also review a few common frequency spans settings: 20, 50, 100, and 200 KHz.
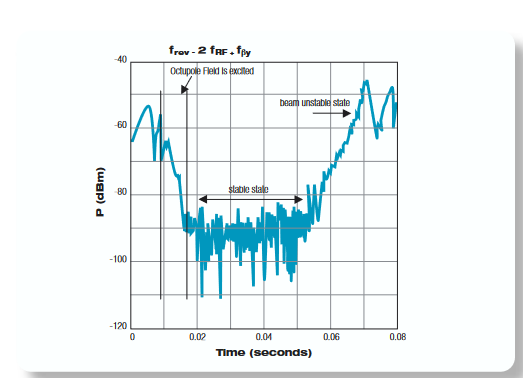
The RSA is able to capture and store up to 25 spectral waveforms in the frequency domain during the typical 160 ms ramp time (156 during the 1 second ramp time). These waveforms can be viewed in either a traditional frequency domain display (as would be seen on a Swept Frequency Analyzer) or in a Spectrogram mode where the X-axis is frequency, Y-axis is time and power is given a color representation.
The RSA provides the ability to recall this data and step back in time through the captured waveforms in 6.4 ms intervals.
Table 1 shows the relationship of frequency span, frame rate, time resolution, and frequency resolution as well as record length (length of events that can be stored in the RSA). The Time resolution is the interval between each of the 1024 samples that makes up the frame.
The RSA can also display the information in a Power vs. Time format with time resolution to 40 nanoseconds and dynamic range superior to an oscilloscope (see Figure 4). How does frequency and time resolution compare with a Swept Spectrum Analyzer (SSA) frequency resolution? Assuming the typical 501 data points of resolution on the SSA display, the answer is shown in Table 2.
Span | Frame Rate | Time Resolution | Frequency Resolution | Record Length RSA3300A (64 MB) | Record Length RSA2200A (2 MB) |
200 KHz | 3.2 ms | 3.125 us | 312.5 Hz | 51.2 sec | 1.6 sec |
100 KHz | 6.4 ms | 6.25 us | 156.25 Hz | 102.4 sec | 3.2 sec |
50 KHz | 12.8 ms | 12.5 us | 78.125 Hz | 204.8 sec | 6.4 sec |
20 KHz | 32 ms | 31.25 us | 331.25 Hz | 512.0 sec | 16.0 sec |
Table 1. Relationship of span, frame time, time resolution, frequency resolution and memory length.
How about sweep time? Here we see the analog and FFT modes offered by some analyzers compared with the Real Time mode of the Tektronix RSA instruments at narrower resolution bandwidth (RBW) settings.
In a swept analyzer, any event that occurs at a particular frequency between the 200 msec it takes to sweep that point will not be observed. The same is true of any event occurring <15 ms in our example FFT instrument of Table 3. The real time capabilities of the Tektronix RSA Series, however, will allow the user to view the entire span in 6.4 ms time slices. In addition, the RSA will allow the user to store and recall these signals for post-capture analysis, a feature not offered in swept spectrum analyzers. The user can recall these signals in “frequency vs. time” or “power versus time” mode with even better time resolution (6.25 us). So we see that the unique architecture and approach offered in the Tektronix RSA2200A and RSA3300A series analyzers provide a definite advantage when measuring transient signals, and often with improved frequency resolution. With fast frame rates, Tektronix real time spectrum analyzers allow for a more dynamic measurement of the chromaticity and application of chromatic correction.
Frequency Span | Swept Analyzer (501 data points) | RSA (Real-time mode) |
100 KHz | 200 Hz | 156 Hz |
20 KHz | 40 Hz | 31 Hz |
Table 2. Comparison of swept analyzer and RSA frequency resolution at 100 KHz and 20 KHz Resolution Bandwidth settings, assuming 501 data points resolution on the swept analyzer.
Analyzer Type | Swept | FFT | Real TIme |
RBW (default) | 1 KHz | 1 KHz | 266.96 Hz |
Sweep Time (Coupled Mode)/Time Resolution | 0.2 sec | 15 ms | 6.4 ms (frame time) 6.25 us (time resolution) |
Table 3. Comparison of time resolution at 100 KHz frequency span between an analog swept spectrum analyzer, an instrument with FFT mode (offered in some analyzers at narrower resolution bandwidth (RBW) settings) and the RSA using Real Time technology. Note the much-improved default RBW setting using the RSA.
Chromaticity measurements
Chromaticity describes the change in betatron resonant frequency (tune) as a function of beam momentum. Chromaticity compensation is required in high-energy storage rings to avoid instability due to the head-tail (H-T) effect. We define the chromaticity of a lattice as Q’ = dQ/dd where Q is the betatron tune and d is the fractional deviation from nominal momentum.
One approach is to measure the coherent damping rates by shock exciting the beam with a fast kicker, then observing the response of beam-position monitor signals on the RTSA. The analyzer displays the damping envelope logarithmically, allowing, after subtraction of the incoherent radiation-damping rate, the determination of the coherent damping rate.
In positron or proton storage rings with many closely spaced bunches, an electron cloud can build up in the vacuum chamber due to photoemission or secondary emission. There then exists the possibility of a single-bunch two- stream instability driven by this electron cloud. Depending on the strength of the beam-electron interaction, the chromaticity, and the synchrotron oscillation frequency, this instability either resembles a linac beam breakup or a head-tail instability.
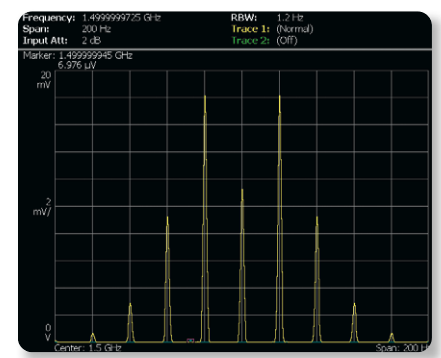

Most tune measurements in the storage ring are made at a single time in the acceleration cycle. However, if the tunes are measured over the entire acceleration cycle, as required by swept frequency analyzers, the process requires many beam cycles making data acquisition extremely laborious and grinding HEP experiments to a halt. The RTSA can acquire and retrieve tune data for the complete acceleration cycle in one beam cycle. It can also be desirable to utilize Phase Modulated RF to measure the chromaticity [3, 4]. The RSA products allow direct Phase Modulation (PM) measurement for faster, more accurate chromaticity calculations (see Figures 5(a) and 5(b)).
Analog Demodulation with Multi-Domain Display
The RSA offers amplitude, frequency and phase demodula- tion capabilities in multi-domain displays. By using marker functions, the RSA makes it possible to easily read out modulation directly on the display (see Figure 6). FM demodulation is useful for a number of HEP applica- tions. In the event of beam instability, for example, it is possible to analyze the variation with high frequency and time resolution (see Figure 7). It is also useful to monitor Betatron signals and for cases where a known amount of external FM is injected into the RF system.
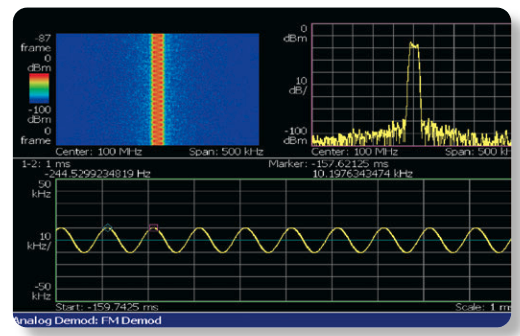
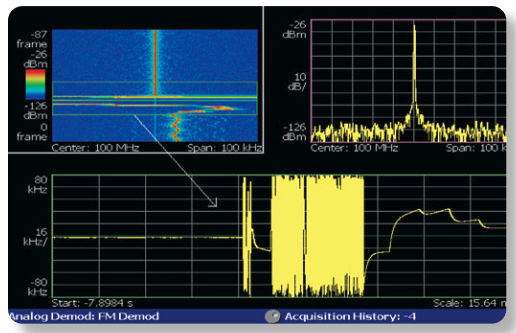
In Figure 7 we see severe signal instability in a multi-mode view. Recall from Table 1 that with a 100 kHz span the Spectrogram (upper left) and Frequency Domain (upper right) views provide time resolution of 6.4 ms and frequency resolution of 156.25 Hz. Using the FM demod mode (bottom), the time resolution (X-axis) can be set to 6.25 us and frequency resolution (Y-axis) to 16 mHz per division, allowing extremely transient and small frequency variations to be observed.
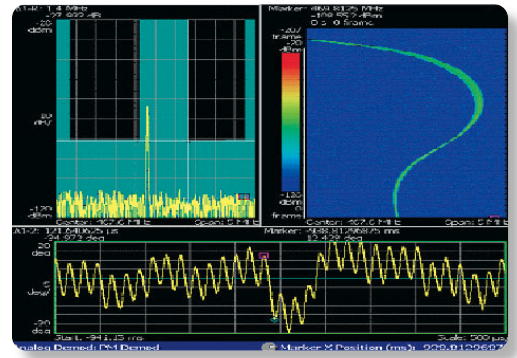
Phase modulation is especially useful to for monitoring phase stability of the beam, for analyzing and adjusting the many Phase Lock Loops (PLL) found in a typical storage ring, and for calculating chromaticity (see Figure 8). Amplitude modula- tion is useful for Synchrotron measurements.
Monitoring Beam Stability with Frequency Mask Trigger
Beam instability appears in the frequency domain as either signals that drift in frequency or as distortion products (see Figure 9).
By using its unique Frequency Mask Trigger (Figure 10), the Tektronix RSA can be set to trigger should either of these events occur. A mask can be “drawn” on the display using a mouse to within one pixel and 0.1 dB of resolution. Once the RSA is triggered, it can begin recording data for later analysis, it can also output a trigger to an external device, such as an alarm. Additionally, the RSA offers a pre-trigger setting, so it is possible to recall and analyze data just prior to the triggering event.
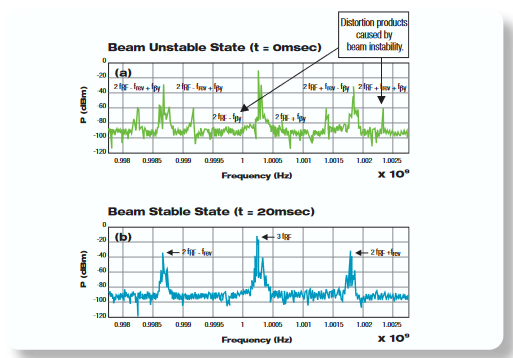
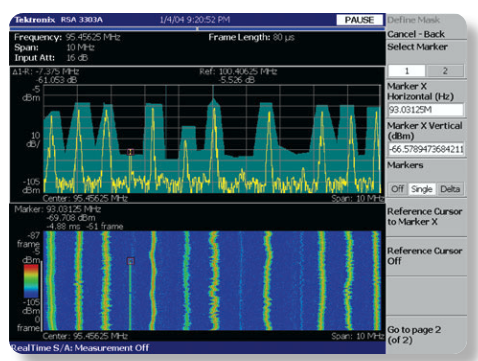
This is a major advantage over the traditional trigger offer- ings found in swept frequency analyzers, which were limited to either a TTL input or an IF level trigger. Swept frequency analyzers monitor the power level of all signals in the IF passband and, consequently, would be constantly triggered on the signal regardless of the distortion product. The RSA3300A offers theses traditional trigger inputs with the additional, unique option of a Frequency Mask Trigger.
Additional Features and Applications
Amplitude Correction
Additional RSA features that can be useful in HEP applications include the ability to input an amplitude correction file with up to 3000 points of frequency response data (see Figure 11). This can be useful for correcting for loss of BPM cables or other losses prior to the RTSA input.
Vibration Analysis
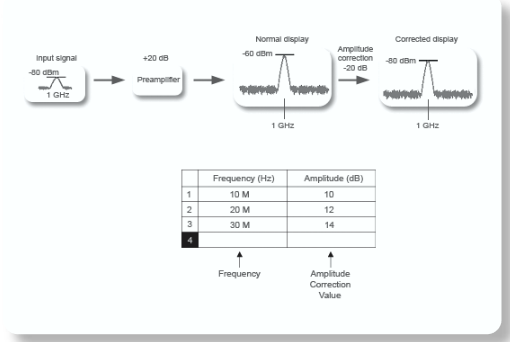
The RSA can be used to measure low frequency vibrations (to DC) with excellent frequency resolution and much better update display rates than traditional swept frequency ana- lyzers. The importance of vibration problems increases with the smaller emittance of a storage ring for synchrotron radi- ation source. Vertical emittance of a storage ring can be so small that the effects of vibration on the electron beam cannot be neglected. Operation of air conditioning, water-cooling and vacuum pumps can cause vibration of the magnets, which then affects beam stability. These vibrations can be analyzed using accelerometers, the output of which can be amplified and fed to the RSA for FFT processing. In addition, intermittent vibration spikes can be captured reliably with the real-time capability of the RSA.
Conclusion
Tektronix real-time spectrum analyzers continue to lead the field in HEP applications by offering a single instrument with capabilities not found in a swept spectrum analyzer:
- Frequency Mask Trigger and Trigger output that allows the user to trigger on a tune frequency at the instant it goes unstable while sending a TTL signal to other instruments (i.e. alarm device) and capturing pre-trigger data
- Capture of long records of seamless data (including pre-trigger data)
- A multi-domain view allowing simultaneous display of any three modes: spectrogram, power vs. time, frequency domain or demodulation data (AM, FM, PM)
- Updated display of the spectrum with better frequency resolution
- Data recall for post-processing and detailed analysis
Tektronix RSA2200A and RSA3300A Series Real-time Spectrum Analyzers deliver unique solutions and advan- tages for operators and researchers in the High Energy Physics field. Only Tektronix real-time spectrum analyzers offer the triggering, capture and analysis features needed to reveal the true nature of particle accelerator anomalies. The RSA is the solution that can go forward with demanding requirements of particle research.
Please contact your local Tektronix representative to request for more information and to arrange for a demonstration of these truly unique instruments.
Find more valuable resources at TEK.COM
Copyright © Tektronix. All rights reserved. Tektronix products are covered by U.S. and foreign patents, issued and pending. Information in this publication supersedes that in all previously published material. Specification and price change privileges reserved. TEKTRONIX and TEK are registered trademarks of Tektronix, Inc. All other trade names referenced are the service marks, trademarks or registered trademarks of their respective companies.
03/04 37W-17749-